
Research Interests
The research focus in Prof. Chuang’s Lab is to understand solid-state materials systematically via computer algorithms and theoretical modelling. This approach includes the use of various levels of total energy calculations, such as force field molecular dynamics, tight-binding molecular dynamics, first-principles calculations, density functional theory, and beyond (GW and hybrid functionals). For a system consisting of more than tens of atoms, a combinatorial approach may be used to solve the problem. However, if the system is too complicated to be solved, genetic algorithms will be used, such as the Wang-Landau algorithm, and the greedy algorithm as tools for materials studies. Machine learning techniques, such as high-throughput and neural networks, are also used to study novel materials. These algorithms were specifically developed to solve various problems encountered in the field of materials physics. A conceptual chart is illustrated below.
Research Areas
2D Topological materials
My expertise in surface systems is very helpful in studying 2D topological insulators, (a.k.a. quantum spin Hall effect), quantum anomalous Hall effect and 2D topological crystalline insulators. I have collaborated with Prof. Hsin Lin at Academia Sinica (formerly the National University of Singapore) and Prof. Arun Bansil at Northeastern University, USA to study the 2D materials in freestanding and upon substrates. We have published more than 14 papers. We published our results in Nano Lett. 14, 2505 (2014) and Nano Lett. 15, 6568 (2015) and npj 2D Materials and Applications 3, 35 (2019).
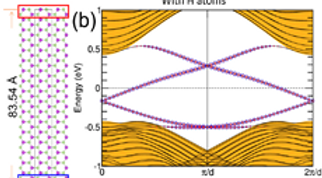
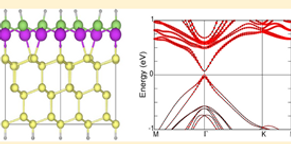
I am the world first prediction of the growth of bismuthene on SiC(0001). I had shown how SiC(0001) substrates can be used to manipulate and tailor the topological properties of Bi and Sb freestanding films via my extensively theoretical proof and calculations. We published our result and let the rest of world to synthesize this bismuthene on SiC(0001). My materials prediction then is confirmed by the work led by Dr. Jörg Schäfer in Universität Würzburg in Germany. The work is titled “Bismuthene on a SiC substrate: A candidate for a high-temperature quantum spin Hall material” and now published in Jun, 2017 [Science 357, 6348 (2017)].
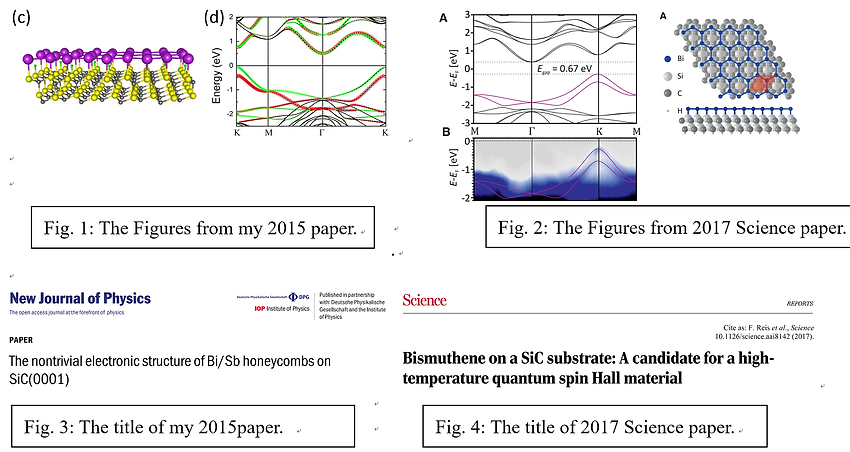
2D magnetic materials and surface of superconductors
We recently teamed up with Prof. M. Zahid Hasan from Princeton University to investigate the impact of single-atom impurities in highly correlated systems. Impurities are excellent tools to probe their intricate and elusive physics. Through our collaborations, we elucidated the unusual suppression of superconductivity induced by Co impurities in the iron-based superconductor, LiFeAs. We have also explored the strong magnetic resonance induced by In impurities in the topological magnet Co3Sn2S2. The results of these studies are published in Physical Review Letters 123 (21), 217004 (2019) and Nature Communications 11, 4415 (2020).
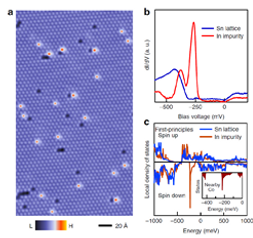
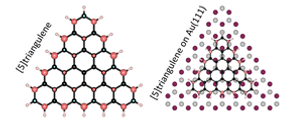
Magnetism is also a highly sought-after property when it comes to developing electronic devices. As devices become smaller and smaller, it also becomes more desirable to develop magnetic materials at a nanoscale level. In our collaboration with Prof. Lu Jiong from the National University of Singapore, we revealed the high-spin ground states of a triangular graphene molecule also known as [5] triangulene. This study is published in Sci Adv 5 (7), eaav7717 (2019).
Metal/Semiconductor and Oxygen/Semiconductor interface

The growth of metals on Si(111) surface has been the focus of many studies over the past several decades. We have studied Au, Ag, Pb, Bi, Sb, and O on primarily silicon substrates. Recently, we proposed the growth of a 2D topological insulator on Si(111) by forming the surface alloy. The experimental collaborators (Prof. Chien-Cheng Kuo, Pin-Ju Hsu (NTHU), Deng-Sung Lin(NTHU) and Prof. Wang-Chi Vincent Yeh (NDHU)) have successfully grown the Bi/Au/Si(111). The result has been published in Phys. Rev. B 93, 035429 (2016) and Phys. Rev. B 98, 121404 (Rapid Communications) (2018).
2D TMD materials
With the discovery of graphene, research regarding 2D materials has gained dominance in recent years. One of the promising candidates is the transition metal dichalcogenides (TMDs), which is defined by a general formula of MX2 with M corresponding to transition metal, and X corresponding to chalcogen S, Se, or Te. The weak interlayer bonding of TMDs facilitates control of film thickness via growth or exfoliation down to submonolayer. As TMDs are reduced to 2D, novel physics often emerges such as indirect to direct band-gap transition, quantum spin Hall effect, strongly enhanced charge density wave (CDW) order, topological surface states, and superconductivity. This breadth of phenomena makes 2D TMDs a promising platform both for the development of next-generation devices and important fundamental studies. My group focuses on elucidating the emerging phenomena during dimensionality cross-over from 3D to the 2D regime.
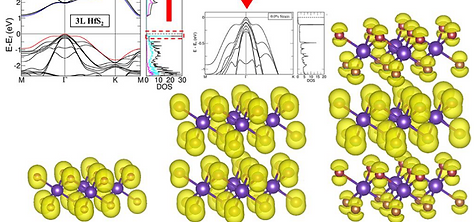
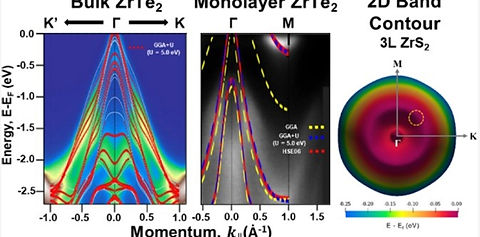
Moreover, my group collaborates with experimental groups, such as Prof. Yu-Lun Chueh (NTHU), Prof. Hao-Chung Kuo (NCTU), and Prof. Tai-Chang Chiang and Prof. Vidya Madhavan (UIUC), to fully realize our theoretical predictions and be utilized for commercial applications.
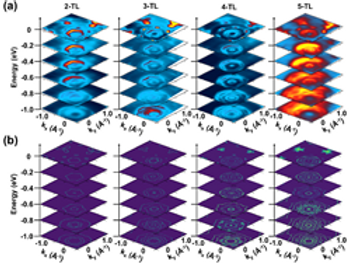
To further extend the exploration of the electronic properties of TMDs, my group investigated their topological properties and Rashba effect through Janus-based structures and functionalization, using Machine Learning techniques, such as the High-Throughput approach.
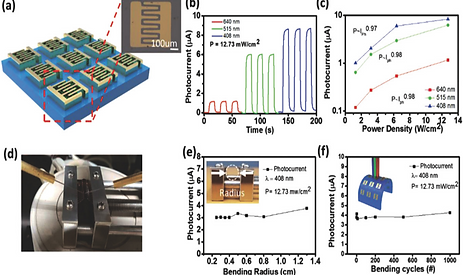
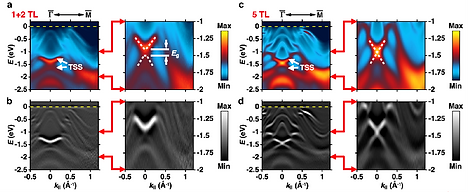
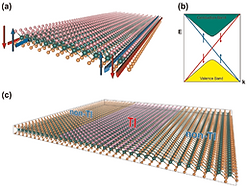
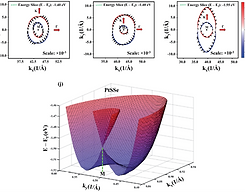
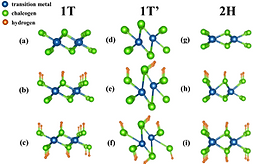
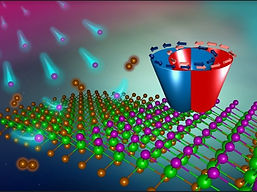
These results have been published in Small 2018, 14, 1800032, npj 2D Materials and Applications (2019) 3:2; ACS Appl. Electron. Mater. 2021, 3, 1071−1079; J. Phys. Chem. C 2021, 125, 1134−1142; New J. Phys. 22 (2020) 053010; npj 2D Materials and Applications (2019) 3:35; Chinese Journal of Physics 66 (2020) 15–23; npj 2D Materials and Applications (2021) 5:40; Phys. Rev. B 102, 115149 (2020); Phys. Rev. Lett. 124, 036402 (2020); and Nanoscale Adv., 2021, Advance Article.
2D MXenes
Two-dimensional (2D) MXenes have been gaining research attention due to their predicted topological phase and promising technological applications. The versatility of the structure and possible functionalization provide possibilities to observe rich physics and tunable electronic and topological properties. Experimental studies show that MXene surfaces are easily terminated by F, O, or OH groups after chemical exfoliation by hydrofluoric acid solutions from the precursor MAX phase. Though few studies have found the topological phases in MXene, here we take a step further and reveal topological phases alongside Zeeman- and Rashba-type splitting which are highly desired properties for spintronics applications. Some of the results are published in Phys. Rev. B 102, 075306 (2020).
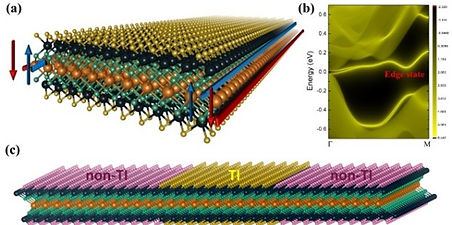
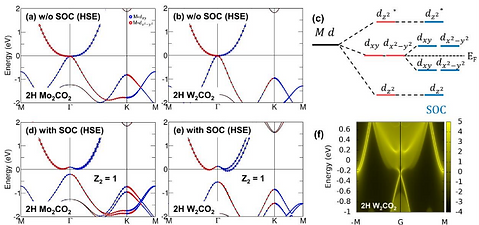
Structural predictions based on Genetic Algorithm and Greedy Algorithm
Computational algorithms are at the tipping point where large parallel computers can effectively serve as the screening phase for finding material compositions and structures with promising “sweet spots” for property optimization. I have participated in the development of genetic algorithms which facilitate studies of systems of atomic clusters, Si surfaces, Si nanowires since my graduate study under Prof. Kai-Ming Ho at Iowa State University. Some of the results have been published in Nano Lett. 2006, 6, 2, 277–281; Phys. Rev. B 69, 165408 (2004); J. Chem. Phys. 127, 144313 (2007); and Surface Science 578 (2005) 183–195.
A. Crystal structure prediction
We developed both genetic algorithms and greedy algorithms to predict the atomic structure of crystals. We have also used the greedy algorithm to predict the structures of superhard bulk materials (e.g., BC2N). Both algorithms were used to predict the structural motifs for the BC2N superlattices. We have retrieved seven structural models for c-BC2N 1×1×1 lattice which were identified previously by Sun et al. [Phys. Rev. B 64, 094108 (2001)]. Furthermore, the atomic structures with the maximum number of C–C bonds for c-BC2N 2×2×2, 3×3×3, and 4×4×4 superlattices were found by imposing the greedy algorithm in the tree data structure. This new structural motif has not been previously proposed in the literature. A total of up to 512 atoms in the c-BC2N superlattice are taken into consideration.

B. Surface reconstructions including Step surfaces (vicinal surfaces) and flat surfaces
The determination of the atomic structure of crystalline surfaces is a long-standing problem in surface science. To solve this problem theoretically, we have developed a genetic algorithm coupled with the silicon empirical potential to search for low energy surface structures. These structures are further optimized using first-principles calculations. STM images of the selected structures are calculated to compare with the experimental data. We have successfully applied this method to several high-index surfaces [e.g. Si(105), Si(114), Si(337), and Si(103)], and continue to study more interesting vicinal semiconductor surfaces.
C. Atomic clusters
Atomic clusters, nanoclusters, or nanoparticles, regarded as a phase of matter, have been studied not only for their interesting physical and chemical properties but also for their future applications. These nanoclusters can form either uniform arrays or nanowires on semiconductor surfaces. The detailed structural study of nanoclusters in the free space is a first step to making the dream of nano-machines feasible. We have studied the electronic properties of the noble metal-doped Si clusters (e.g. Au, Ag, and Cu). Recently, we study the binary atomic clusters which can be used for hydrogen storage. The genetic algorithm coupled with first-principles calculations were used to search for the atomic structures of nanoclusters.

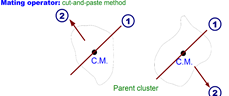
Energy, evolution reactions, thermoelectric,
and database-driven related projects
Energy technology is one of the most urgent technologies in the world nowadays. In August 2009, the Office of Basic Energy Sciences in the U.S. Department of Energy’s Office of Science established 46 Energy Frontier Research Centers (EFRCs). I have secured an International Collaborative proposal from NSC of Taiwan to participate in one of EFRCs established in UCLA and focused on two research topics. One is to study the dynamic process of hydrogen storage at the interface between the bulk materials and its decomposed product alloy. The other is to predict the new alloy materials with a higher thermoelectric figure of merit for thermoelectric applications. Both directions use either the first-principles calculations or simple force field method coupled with different search algorithms (genetic algorithm and Wang-Landau algorithm) to determine the atomic and electronic structures.
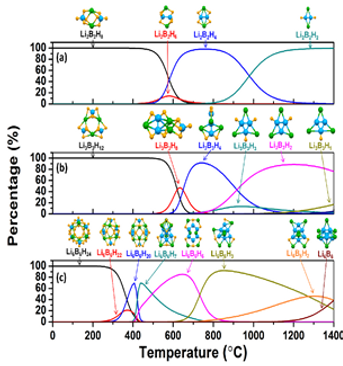
Supplying the world’s growing population with clean, affordable energy is a critical challenge. However, the challenges of energy storage and integration into the current power grid have hindered the implementation of these intermittent renewable energy sources. Electrochemical and photoelectrochemical water splitting are promising ways to store sustainable, intermittent energy resources (e.g., wind and solar) in the form of hydrogen, an energy vector with high energy density and potentially without carbon emissions. Oxygen evolution reaction (OER) and hydrogen evolution reaction (HER) play a key role in water splitting and carbon dioxide reduction processes. However, due to the intrinsic sluggish kinetics originating from a series of proton-coupled electron transfer steps, there are still substantial challenges encountered during the search for robust and cost-efficient OER and HER electrocatalysts. Therefore, exploring the promising alternative electrocatalysts is of great interest to the community. Thus, we performed OER and HER calculations in collaboration with experimental groups from the City University of Hong Kong and National Tsing-Hua University. Some of the results have been published in ACS Applied Materials & Interfaces 11, 33012 (2019).
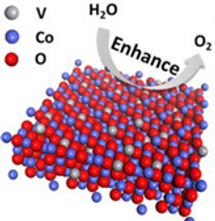
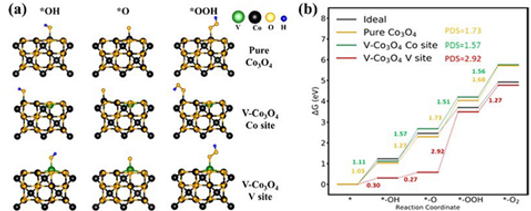
Finally, we are also involved in other energy-related studies such as thermoelectric search and database-driven materials search.
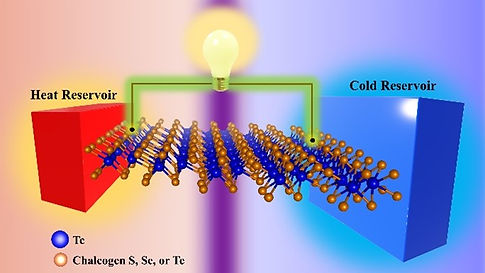
